Re-evaluation and operative indications after induction therapy for thymic epithelial tumors
Introduction
Thymic epithelial tumors (TETs), including thymoma and thymic carcinoma, are rare, with an incidence rate of approximately 1.3–3.2 cases per million (1). Surgical resection is the primary treatment approach for stage I–III TETs; however, complete resection can be challenging in cases of locally advanced TETs. In such cases, preoperative induction therapies have been explored to achieve complete resection. Various chemotherapeutic regimens have been utilized, including adriamycin and/or platinum-based multiagent combinations (2). If remarkable tumor shrinkage occurs, extensive surgery should be offered, and postoperative radiotherapy might be considered. Conversely, in patients with unresectable tumor after systemic induction treatment, concomitant chemoradiotherapy may be considered (3,4). The latest National Comprehensive Cancer Network guidelines recommend induction chemotherapy for patients with potentially resectable locally advanced TETs (5). A significant clinical challenge lies in assessing potential resectability at two critical time points: before induction therapy (or any treatment) and after induction therapy but before surgery. Re-evaluation is important because it is related with the decision for an invasive surgery after burdensome systemic therapy. This review specifically focuses on the re-evaluation process and operative indications following induction therapy for TETs.
While recognizing the distinct oncological and clinical behaviors of thymoma and thymic carcinoma, the limited evidence in this field necessitates a discussion of these issues under the broader category of TETs, including both thymoma and thymic carcinoma. Where possible, we have endeavored to discuss them separately, acknowledging the nuances between the two.
This review focuses on the re-evaluation process and operative indications following induction therapy for TETs.
Re-evaluation methods
Computed tomography (CT) scan
In the re-evaluation of TETs after induction therapy as well as in the primary evaluation (6), CT is the reference standard for radiological evaluation (7). CT is primarily used to evaluate therapeutic effects of induction therapy. Measuring the tumor response to induction therapy is important for both clinical decision-making and in the setting of multi-institutional studies (8). Among various methods, the Response Evaluation Criteria In Solid Tumors (RECIST) version 1.1 is most commonly used, and the International Thymic Malignancy Interest Group (ITMIG) has suggested using a practical guide for standard outcome measures of TETs (8,9). The RECIST criteria for TETs will be described below.
CT is also performed to assess resectability. Locally advanced TETs extend into the mediastinum and thoracic cavity and can encompass nearby structures (10). Although CT results cannot reveal a tumor cell-free border, it can still provide hints that might support performing complete resection. Particularly in contrast-enhanced CT scans, there is a potential to somewhat delineate the boundaries between the tumor and surrounding structures due to the contrast. According to a prospective study for thymoma conducted by Shen et al., lobulated or irregular tumor shape, unsmooth contour, heterogeneous nature, heterogeneous enhancement pattern, and invasion of adjacent structures were found to be related to incomplete resection in univariable analysis (11). Subsequent multivariable logistic regression showed that only absence of arterial system invasion was a significant factor supportive of complete resection. Similarly, in a study by Hayes et al., the factors related with incomplete resection of thymoma included a lobulated tumor contour, ≥50% abutment of the circumference of an adjacent vessel, thoracic lymphadenopathy, adjacent lung changes, and pleural nodularity (12). Tumor size was larger in the incompletely resected group than in the completely resected group. The multivariable analysis performed by Hayes et al. revealed that ≥50% abutment of the circumference of an adjacent vessel and pleural nodularity were independent predictors of incomplete resection. In the case of thymic carcinoma, Hayes et al. also found that incomplete resection was associated with tumors contacting >25% of an adjacent mediastinal structure and a tumor size of >7.5 cm (13). Furthermore, other radiographic characteristics associated with advanced stages of TETs consist of tumor sizes of ≥7 cm, a lobulated tumor contour, mediastinal fat infiltration, and an elevated hemidiaphragm (14,15). In recent years, evidence has emerged suggesting that the size of TETs plays a role in patient prognosis with several papers published assessing the impact of tumor size on survival (16). Additionally, the International Association for the Study of Lung Cancer Thymic Epithelial Tumor Staging Project recently proposed the ninth edition of the Tumor-Node-Metastasis (TNM) Classification of Malignant Tumors. This new classification includes the division of the T1 category into T1a (≤5 cm) and T1b (>5 cm) (17). However, there has been a debate regarding the size threshold for predicting incomplete resection status or advanced stage because some larger thymomas can be noninvasive and fully resected upfront in asymptomatic patients (18). Furthermore, the necessity for phrenic nerve resection is challenging to predict solely based on imaging in the absence of an elevated hemidiaphragm, presenting an ongoing challenge for surgeons (19).
Magnetic resonance imaging (MRI)
MRI can be used in addition to CT for better evaluation of the tumor composition and in patients with suspicious tumor infiltration of adjacent structures (6,20). Particularly, cystic or necrotic parts combined with inhomogeneous contrast-enhancement strongly suggest invasiveness (21,22). However, MRI is incapable of precisely detecting low-level invasiveness. To overcome this problem, MRI with cine sequences may show sliding motions between the tumor and cardiovascular structures and might provide more reliable tumor invasion detection (23).
Positron emission tomography-CT (PET-CT)
Encouraging results showing the prognostic ability of PET-CT after induction therapy have been published, although the reports were from studies with a small number of patients (24). In a study with fourteen patients, Fukumoto et al. have documented a noteworthy decline in the standardized uptake value (SUV)max in six patients exhibiting an Ef2 response. Conversely, in 5 out of 8 patients with an Ef0–Ef1 response, there was a decrease in SUVmax, although it did not reach statistical significance (25). Korst et al. also suggested the effectiveness of 18F-fluorodeoxyglucose (18F-FDG) PET-CT for tumor response assessment after induction therapy (26). Similarly, Matsumoto et al. reported the effectiveness of 18F-FDG PET-CT for assessing the therapeutic efficacy in five patients with increased pathological responses associated with larger decreases in SUVmax (27).
Tumor markers
Although there are no specific tumor markers for thymoma, some are practically available for thymic carcinoma. A recent multi-institutional retrospective study on advanced thymic carcinoma has revealed significant insights into these markers. According to the NEJ 023 study (28), serum neuron-specific enolase (NSE) was identified as a potential prognostic tumor marker for advanced thymic carcinoma across various histological subtypes. Additionally, squamous cell carcinoma (SCC) antigen levels have shown promise as prognostic indicators in patients with thymic SCC. In fact, a case report involving a patient with thymic small cell carcinoma who underwent multimodal therapy revealed that NSE levels partly reflected tumor progression and treatment responsiveness (29). Another study highlighted the diagnostic potential of the cytokeratin 19 fragment (CYFRA 21-1) in thymic SCC (30). However, this marker was not found to be associated with overall survival or progression-free survival in a separate study (28). This discrepancy underscores the need for further investigation into the prognostic value of CYFRA 21-1. Therefore, for cases of advanced thymic carcinomas, it can be reasonably inferred that during the re-evaluation phase, measuring tumor markers has the potential to offer valuable insights for evaluating the therapeutic efficacy of initial treatment and making well-informed decisions regarding subsequent interventions.
Pathological examination
In the context of the re-evaluation, the role played by pathological examination may not be extensive. In fact, re-biopsy for TETs is infrequently performed. This procedure is typically considered when induction treatment proves ineffective in achieving the desired outcomes, and tumor regrowth is observed. A possible purpose of re-biopsy is to modify the treatment for the next therapeutic session (31,32).
On the other hand, pathological findings after the primary treatment provide valuable treatment-related information and insights into prognosis. Johnson et al. introduced a concept of tumor response grade (TRG), proposed by Mandard et al., into TETs (33,34). Pathological tumor response after induction therapy is graded from 1 to 5 based on necrosis and viable tumor cells (33-35). Johnson et al. reported that TRG for TETs appeared reproducible and correlated with the radiologic response of tumor size. Moreover, Wang et al. identified TRG as one of the independent prognostic factors, alongside ypTNM, ypMasaoka, and complete resection, through multivariable analysis in a cohort of 81 patients with TETs undergoing induction therapy (35). Of note, clinical downstaging (c > yc) of TNM or Masaoka classifications did not change the pooled hazard ratio for survival in their study. This implies an interesting notion that pathological evaluation with TRG might be more accurate in predicting prognosis than other radiological evaluations in the re-assessment phase.
Therefore, while re-biopsy might be considered important in cases requiring careful evaluation for surgery after neoadjuvant treatment in TETs, its effectiveness in guiding surgical decisions remains unproven. Similarly to TETs, although biopsy-guided pathological response assessment in cancers such as breast cancer and melanoma has shown promise, it has not been widely adopted in clinical practice due to a lack of conclusive evidence. This underscores the universal challenge in establishing the clinical utility of biopsy-based prognostic evaluations across different types of cancers.
Assessment of treatment response
The timing for the assessment
Surgical resection is typically considered 4 to 8 weeks after induction therapy, assuming patients are deemed to have sufficiently recovered (36,37). If delayed further, the efficacy of the induction therapy may diminish. Therefore, although there are no specific guidelines on the timing for reassessing the initial treatment’s effectiveness, some literature suggests a window of 2 to 4 weeks after completing preoperative therapy (36).
Evaluation based on RECIST criteria
To evaluate the change in tumor burden after treatment, the ITMIG suggests using the modified RECIST criteria to perform a standardized assessment of the treatment response of TETs (8). Currently, the most commonly used method is RECIST version 1.1, which is basically a unidimensional tumor measurement. ITMIG recommends RECIST version 1.1 for response assessment, but with certain caveats and modifications (38). First, pretreatment and post-treatment imaging should be interpreted by the same individual. Second, because TETs tend to spread along the pleura, the RECIST version 1.1 criteria may not be ideal. Therefore, ITMIG recommends adhering to the criteria previously established for pleural measurements in mesothelioma, measuring the short axis of the tumor.
According to a meta-analysis, four studies have reported analyses correlating the completeness of resection with responses to induction therapy measured by RECIST criteria. (26,39-42). Collectively, these studies included a total of 105 patients. One study employed induction chemoradiotherapy with a radiation dose of 45 Gy, whereas the remaining studies focused solely on induction chemotherapy. All studies utilized cisplatin-based regimens. The pooled odds ratio assessed in the meta-analysis favored a response to induction therapy predicting complete resection, with a value of 1.4 (95% confidence interval: 0.49 to 4.0; P=0.53). Although the result did not reach statistical significance, the assessment by the RECIST criteria remains an essential tool to evaluate the therapeutic efficacy of induction therapy for TETs.
Re-staging
Theoretically, benefits of induction therapy lie in the downstaging of advanced TETs. However, according to Wang et al.’s retrospective study, clinical Masaoka downstaging was only observed in 24.7% of patients, while 46.9% experienced clinical TNM downstaging (35). They also noted that clinical outcomes were similar between patients who underwent clinical downstaging post induction therapy and those who did not. Whether it was the clinical downstaging of Masaoka, TNM, T, N, or M classifications, these factors didn’t stand as independent prognostic variables. The authors suggested that assessing clinical re-staging was challenging due to other biases. Therefore, a possibility of underestimating clinical downstaging might be considered.
Evaluation beyond oncological aspects
Induction therapy, such as chemotherapy and radiation, may lead to a decline in the patient’s overall health, potentially affecting their physical fitness for surgery. The treatment can also result in the impairment of specific organ functions, such as the heart, liver, kidneys, and lungs, which can impact the patient’s ability to undergo surgical procedures. Thus, some patients may become ineligible for the intended surgical procedures after undergoing induction therapy, which raises the challenge of determining alternative treatment options. Therefore, assessing a patient’s surgical tolerance after induction therapy is crucial to ensure that they can withstand the rigors of surgery (43).
When there is infiltration into the cardiovascular system, a more meticulous evaluation is required to assess the need for cardiopulmonary bypass and to determine the feasibility of surgical intervention (44). Close collaboration with cardiothoracic surgeons, anesthesiologists, and the cardiopulmonary bypass team is indispensable in such cases. In situations where patients have concomitant neurological disorders like severe myasthenia gravis, it is necessary to coordinate with the neurology department for an evaluation of the specific condition. Even if no crisis is evident at the time of evaluation, it is imperative to anticipate the possibility of post-operative crises and engage in discussions involving anesthesiologists, neurologists, and intensive care unit physicians (45).
Surgical indication after induction therapy
Complete resection
The primary goal of induction therapy in TETs, as reiterated, is to achieve complete resection by reducing the tumor, inducing pathological changes in the tumor, downstaging, and other alterations, following the primary treatment. This is because achieving a complete resection after induction therapy may represent a significant prognostic factor or indicate a tendency for improved overall survival (39,41,46-48).
The final decision is typically entrusted to the surgeon’s judgment regarding the invasiveness of TETs and the possibility of complete resection (19). However, determining the feasibility of complete resection is not a straightforward task. Hence, objective assessments using imaging studies, as mentioned earlier, become crucial. An analysis of the Japanese Association for Research on the Thymus database revealed that in patients with TETs infiltrating major vessels, the rate of complete resection was lower than that in patients with TET infiltration into the pericardium or lungs (49). In fact, resection of small wedge-shaped portions of the lung adjacent to the pericardium essentially does not increase morbidity associated with thymectomy and almost always achieves negative margins in these areas. Contrarily, vascular resections are far more complex and are associated with higher morbidity; therefore, surgeons outside high-volume centers may hesitate to perform such resections (19).
Considering the surgical approach is crucial. To achieve optimal exposure, the standard sternotomy is generally employed, with the addition of more extensive approaches such as the hemiclamshell, clamshell, or even the transmanubrial osteomuscular-sparing approach (50,51). Furthermore, video-assisted procedures can complement open thoracotomy to ensure an adequate surgical view (51). Recent reports have highlighted the successful application of minimally invasive approaches exclusively for the surgery of advanced TETs. Taken together, the choice of surgical approach should be individually tailored based on the tumor’s location, degree of infiltration, response to initial treatment, patient-specific factors, and the surgeon’s expertise (52,53).
Debulking surgery
Even if achieving complete resection is not feasible, debulking surgery or volume reduction surgery yields certain therapeutic effects. A meta-analysis demonstrated improved overall survival in patients undergoing debulking surgery for unresectable thymoma with radical intent compared to those undergoing surgical biopsy (54). Similarly, in cases of thymic cancer, maximal debulking surgery might be beneficial and warrant evaluation for advanced diseases where complete resection is challenging, as indicated by a nationwide database in Japan (55). Other researchers have suggested that combining debulking surgery with radiation could be a treatment option for unresectable TETs (56,57). Recently, Mastromarino et al. presented an intriguing study on unradical surgery for locally-advanced thymoma (58). In their research, patients who underwent incomplete resection followed by adjuvant treatments exhibited similar cancer-specific survival and overall survival rates to those who underwent complete resection in stage III–IVa thymomas. Certainly, the utility of debulking surgery requires further validation through prospective studies or similar research. Nevertheless, in scenarios where complete surgical removal of the tumor is not possible following initial treatments, debulking surgery could be considered as a viable treatment strategy.
Cessation of surgery after induction therapy
In certain cases, due to the morbidity associated with induction therapy and the potential for treatment-related complications or disease progression, secondary resection is not performed. The rate of surgery cessation after induction therapy varies widely, ranging from 4.8% to 38.1% (41,59-61). In these instances, induction therapy might unnecessarily delay or hinder curative resection, potentially allowing disease progression (60). Hence, when there exists a high level of confidence in the potential for achieving complete resection, opting for upfront surgical resection can be considered. Additionally, patients with a compromised performance status or comorbidities that might worsen due to chemotherapy or similar treatments might also be candidates for a surgical approach. As noted in a previous section, debulking surgery yields certain therapeutic effects.
In any case, the indication for induction therapy remains a significant issue to consider. Understanding such high-risk features that may hinder complete resection should help healthcare providers appropriately select patients for induction therapy. Candidate selection for induction therapy should be performed by a multidisciplinary oncology committee that includes oncologists, diagnostic radiologists, surgeons, therapeutic radiologists, and pathologists with experience in managing advanced thymic malignancies (62).
Future perspective in re-evaluation of induction therapy for TETs
Advancements in imaging techniques for accurate re-evaluation
In recent years, the field of radiology has witnessed remarkable advancements in imaging techniques, and their application in the re-evaluation of TETs has been particularly promising. High-resolution CT and PET-CT have become indispensable tools for precise disease evaluation after induction therapy. The integration of artificial intelligence and machine-learning algorithms into image interpretation has further improved the accuracy of identifying resectability of TETs (63-65). The development of more specific radiotracers and functional imaging modalities has offered enhanced sensitivity for detecting small residual lesions and assessing their metabolic activity. These advancements provide a foundation for more informed clinical decision-making, which enables selecting tailored therapeutic strategies based on the extent and characteristics of any remaining disease.
Incorporation of molecular and genetic markers for personalized treatment strategies
Recent advancements in precision medicine can now be applied to TETs (66). The programmed cell death ligand-1 (PD-L1) expression and the tumor mutational burden, biomarkers for response to immunotherapy, are studied well in this field (67). Although promising response rates and survival outcomes have been observed, the application of immunotherapy in TETs is complicated by the occurrence of severe autoimmune-related adverse events, highlighting the need for careful patient selection and management. Further research is essential to identify reliable predictive markers and to optimize the balance between efficacy and toxicity. Moreover, recent research has led to the identification of specific molecular alterations associated with TETs, such as GFT2I, HRAS, NRAS, TP53, c-KIT, EGFR and IGFR (68-70).
Then, the integration of molecular and genetic markers into re-evaluation is a promising approach. The identification of specific genetic alterations and biomarkers associated with TETs can guide tailored therapeutic approaches with the emergence of next-generation sequencing technologies, we can now delve into a tumor’s genomic landscape, identifying mutations and alterations that might influence the treatment response. For instance, research into activating mutations in the KIT oncogene, found in approximately 5% of thymic carcinomas, has been pursued as a potential therapeutic target. Tyrosine kinase inhibitors such as imatinib and sunitinib, which target these mutations, have shown promising results in certain patients, according to studies (71,72).
Incorporation of these markers into re-evaluation enables assessment of treatment effectiveness and development of personalized treatment strategies. This molecular insight not only aids in the identification of targetable alterations but also offers valuable prognostic information that will help clinicians optimize the management of TETs.
Prospects of targeted therapies in the neoadjuvant setting
Targeted therapies have revolutionized cancer treatment, and their potential application in the neoadjuvant setting for TETs is an exciting new area (66). By understanding the genetic and molecular profiles of a patient’s tumor through re-evaluation, clinicians can tailor neoadjuvant therapies to target specific pathways or mutations. Targeted agents, such as tyrosine kinase inhibitors and immune checkpoint inhibitors, hold promise in improving treatment responses and minimizing surgical complications. Early-phase clinical trials investigating the neoadjuvant use of these agents are underway with the aim of assessing their effect on disease downstaging, treatment tolerability, and long-term outcomes. As we gain a deeper understanding of the biological underpinnings of TETs, the integration of targeted therapies in the neoadjuvant context potentially can reshape the landscape of treatment strategies for these rare malignancies.
Discussion
One of the key limitations of this review article is the relatively limited number of published studies and clinical trials in the field of TETs induction therapy and re-evaluation. The scarcity of high-quality, comprehensive data has made it challenging to draw robust conclusions. Our perspective, to a certain extent, was derived from the existing body of knowledge on TETs. Additionally, the heterogeneity in TETs’ subtypes, treatment approaches, and evaluation systems across different institutions and regions has hindered our ability to perform a meta-analysis or provide a uniform, evidence-based guideline for re-evaluation protocols. Nevertheless, this review primarily explores current practices through the lens of available literature. We believe that by accumulating and discussing current clinical experiences and practices, this review can pave the way for future recommendations on how to proceed in the face of such evidence gaps.
Furthermore, another significant issue was our consolidation of discussions on thymoma and thymic carcinoma under the single umbrella of TETs, despite their distinct oncological and clinical profiles. This amalgamation was driven by the extreme scarcity of re-evaluation literature specific to each type, compelling us to address them as a collective entity. However, it is important to acknowledge that there are many commonalities between these two histological types, allowing for a degree of discussion from a comprehensive perspective. Where possible, we endeavored to discuss them separately, acknowledging the nuances between the two.
On the other hand, a notable strength of this review is its pioneering approach to the topic of TETs induction therapy and re-evaluation. To the best of our knowledge, no previous review has comprehensively addressed the specific challenges and opportunities related to re-evaluation in the context of TETs management. We consolidated existing knowledge and provided insights into this crucial aspect of TETs’ treatment demonstrates. While the limitations mentioned earlier exist, our review incorporates the available evidence and provides a synthesis of the current state of knowledge. By doing so, we aim to guide clinicians in their decision-making processes and inspire additional research efforts that may eventually enhance our understanding of the re-evaluation and its implications for patient care.
Conclusions
We have conducted a review of induction therapy and re-evaluation for TETs. Figure 1 shows a flow chart of re-evaluation after induction therapy for TETs. In practical terms, the decision-making process for surgery following induction therapy relies heavily on the empirical judgment of practicing surgeons. Consequently, our conclusion underscores the need for further data accumulation on this subject. If any guidance can be derived from existing retrospective case series, the next logical step should involve conducting randomized prospective trials across multiple institutions. Another critical aspect to address is the attempt to reduce the instances of failure to progress to surgery despite undergoing induction therapy. To this end, it is imperative to prioritize careful patient selection for initial treatment. The candidate selection for the treatment should be performed by a multidisciplinary oncology committee that includes oncologists, surgeons, and radiation oncologists with experience in managing advanced TETs.
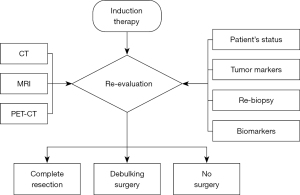
Acknowledgments
Funding: None.
Footnote
Provenance and Peer Review: This article was commissioned by the editorial office, Mediastinum for the series “Locally Advanced Thymic Epithelial Tumors”. The article has undergone external peer review.
Peer Review File: Available at https://med.amegroups.com/article/view/10.21037/med-23-70/prf
Conflicts of Interest: All authors have completed the ICMJE uniform disclosure form (available at https://med.amegroups.com/article/view/10.21037/med-23-70/coif). The series “Locally Advanced Thymic Epithelial Tumors” was commissioned by the editorial office without any funding or sponsorship. M.H. served as the unpaid Guest Editor of the series and serves as an unpaid editorial board member of Mediastinum from May 2022 to December 2025. The authors have no other conflicts of interest to declare.
Ethical Statement: The authors are accountable for all aspects of the work in ensuring that questions related to the accuracy or integrity of any part of the work are appropriately investigated and resolved.
Open Access Statement: This is an Open Access article distributed in accordance with the Creative Commons Attribution-NonCommercial-NoDerivs 4.0 International License (CC BY-NC-ND 4.0), which permits the non-commercial replication and distribution of the article with the strict proviso that no changes or edits are made and the original work is properly cited (including links to both the formal publication through the relevant DOI and the license). See: https://creativecommons.org/licenses/by-nc-nd/4.0/.
References
- Engels EA, Pfeiffer RM. Malignant thymoma in the United States: demographic patterns in incidence and associations with subsequent malignancies. Int J Cancer 2003;105:546-51. [Crossref] [PubMed]
- Ettinger DS, Riely GJ, Akerley W, et al. Thymomas and thymic carcinomas: Clinical Practice Guidelines in Oncology. J Natl Compr Canc Netw 2013;11:562-76. [Crossref] [PubMed]
- Girard N, Lal R, Wakelee H, et al. Chemotherapy definitions and policies for thymic malignancies. J Thorac Oncol 2011;6:S1749-55. [Crossref] [PubMed]
- Girard N, Ruffini E, Marx A, et al. Thymic epithelial tumours: ESMO Clinical Practice Guidelines for diagnosis, treatment and follow-up. Ann Oncol 2015;26:v40-55. [Crossref] [PubMed]
- NCCN Clinical Practice Guidelines in Oncology. Thymomas and Thymic Carcinomas. Version 2. 2022. Available online: http://www.nccn.org/professionals/physician_gls/PDF/thymic.pdf (accessed August 15, 2023).
- Ried M, Marx A, Götz A, et al. State of the art: diagnostic tools and innovative therapies for treatment of advanced thymoma and thymic carcinoma. Eur J Cardiothorac Surg 2016;49:1545-52. [Crossref] [PubMed]
- Filosso PL, Guerrera F, Sandri A, et al. Multimodality therapy for locally-advanced thymic epithelial tumors: where are we now? J Thorac Dis 2016;8:1428-30. [Crossref] [PubMed]
- Benveniste MF, Korst RJ, Rajan A, et al. A practical guide from the International Thymic Malignancy Interest Group (ITMIG) regarding the radiographic assessment of treatment response of thymic epithelial tumors using modified RECIST criteria. J Thorac Oncol 2014;9:S119-24. [Crossref] [PubMed]
- Eisenhauer EA, Therasse P, Bogaerts J, et al. New response evaluation criteria in solid tumours: revised RECIST guideline (version 1.1). Eur J Cancer 2009;45:228-47. [Crossref] [PubMed]
- Zhang Y, Lin D, Aramini B, et al. Thymoma and Thymic Carcinoma: Surgical Resection and Multidisciplinary Treatment. Cancers (Basel) 2023;15:1953. [Crossref] [PubMed]
- Shen Y, Gu Z, Ye J, et al. CT staging and preoperative assessment of resectability for thymic epithelial tumors. J Thorac Dis 2016;8:646-55. [Crossref] [PubMed]
- Hayes SA, Huang J, Plodkowski AJ, et al. Preoperative computed tomography findings predict surgical resectability of thymoma. J Thorac Oncol 2014;9:1023-30. [Crossref] [PubMed]
- Hayes SA, Huang J, Golia Pernicka J, et al. Radiographic Predictors of Resectability in Thymic Carcinoma. Ann Thorac Surg 2018;106:242-8. [Crossref] [PubMed]
- Marom EM, Milito MA, Moran CA, et al. Computed tomography findings predicting invasiveness of thymoma. J Thorac Oncol 2011;6:1274-81. [Crossref] [PubMed]
- Padda SK, Terrone D, Tian L, et al. Computed Tomography Features associated With the Eighth Edition TNM Stage Classification for Thymic Epithelial Tumors. J Thorac Imaging 2018;33:176-83.
- Cangir AK, Yenigün BM, Direk T, et al. Different View on Tumor Size Dilemma in Tumor-Node-Metastasis Staging System for Thymoma. Thorac Cardiovasc Surg 2021;69:148-56. [Crossref] [PubMed]
- Okumura M, Marino M, Cilento V, et al. The International Association for the Study of Lung Cancer Thymic Epithelial Tumor Staging Project: Proposal for the T Component for the Forthcoming (Ninth) Edition of the TNM Classification of Malignant Tumors. J Thorac Oncol 2023;18:1638-54. [Crossref] [PubMed]
- Burt BM, Yao X, Shrager J, et al. Determinants of Complete Resection of Thymoma by Minimally Invasive and Open Thymectomy: Analysis of an International Registry. J Thorac Oncol 2017;12:129-36. [Crossref] [PubMed]
- Patel DC, Shrager JB, Padda SK. The role of induction therapy for thymic malignancies: a narrative review. Mediastinum 2020;4:36. [Crossref] [PubMed]
- Marom EM. Imaging thymoma. J Thorac Oncol 2010;5:S296-303. [Crossref] [PubMed]
- Marom EM. Advances in thymoma imaging. J Thorac Imaging 2013;28:69-80; quiz 81-3. [Crossref] [PubMed]
- Restrepo CS, Pandit M, Rojas IC, et al. Imaging findings of expansile lesions of the thymus. Curr Probl Diagn Radiol 2005;34:22-34. [Crossref] [PubMed]
- Seo JS, Kim YJ, Choi BW, et al. Usefulness of magnetic resonance imaging for evaluation of cardiovascular invasion: evaluation of sliding motion between thoracic mass and adjacent structures on cine MR images. J Magn Reson Imaging 2005;22:234-41. [Crossref] [PubMed]
- Lococo F, Chiappetta M, Triumbari EKA, et al. Current Roles of PET/CT in Thymic Epithelial Tumours: Which Evidences and Which Prospects? A Pictorial Review. Cancers (Basel) 2021;13:6091. [Crossref] [PubMed]
- Fukumoto K, Fukui T, Okasaka T, et al. The Role of (18)F-fluorodeoxyglucose Positron Emission Tomography-Computed Tomography for Predicting Pathologic Response After Induction Therapy for Thymic Epithelial Tumors. World J Surg 2017;41:1828-33. [Crossref] [PubMed]
- Korst RJ, Bezjak A, Blackmon S, et al. Neoadjuvant chemoradiotherapy for locally advanced thymic tumors: a phase II, multi-institutional clinical trial. J Thorac Cardiovasc Surg 2014;147:36-44, 46.e1.
- Matsumoto I, Oda M, Takizawa M, et al. Usefulness of fluorine-18 fluorodeoxyglucose-positron emission tomography in management strategy for thymic epithelial tumors. Ann Thorac Surg 2013;95:305-10. [Crossref] [PubMed]
- Mimori T, Shukuya T, Ko R, et al. Clinical Significance of Tumor Markers for Advanced Thymic Carcinoma: A Retrospective Analysis from the NEJ023 Study. Cancers (Basel) 2022;14:331. [Crossref] [PubMed]
- Terada J, Toyoda Y, Takeuchi E, et al. Surgical resection combined with perioperative chemotherapy for a patient with locally recurrent, previously stage IV thymic small-cell carcinoma: A case report. Thorac Cancer 2022;13:3415-9. [Crossref] [PubMed]
- Shiiya H, Ujiie H, Hida Y, et al. Elevated serum CYFRA 21-1 level as a diagnostic marker for thymic carcinoma. Thorac Cancer 2021;12:2933-42. [Crossref] [PubMed]
- Kobayashi M, Funaki S, Fukui E, et al. A case of alpha-fetoprotein-positive thymic small cell carcinoma: a case report. Surg Case Rep 2023;9:165. [Crossref] [PubMed]
- Girard N. Chemotherapy and targeted agents for thymic malignancies. Expert Rev Anticancer Ther 2012;12:685-95. [Crossref] [PubMed]
- Johnson GB, Aubry MC, Yi ES, et al. Radiologic Response to Neoadjuvant Treatment Predicts Histologic Response in Thymic Epithelial Tumors. J Thorac Oncol 2017;12:354-67. [Crossref] [PubMed]
- Mandard AM, Dalibard F, Mandard JC, et al. Pathologic assessment of tumor regression after preoperative chemoradiotherapy of esophageal carcinoma. Clinicopathologic correlations. Cancer 1994;73:2680-6. [Crossref] [PubMed]
- Wang S, Jiang J, Gao J, et al. Induction Therapy Followed by Surgery for Unresectable Thymic Epithelial Tumours. Front Oncol 2021;11:791647. [Crossref] [PubMed]
- Wright CD, Choi NC, Wain JC, et al. Induction chemoradiotherapy followed by resection for locally advanced Masaoka stage III and IVA thymic tumors. Ann Thorac Surg 2008;85:385-9. [Crossref] [PubMed]
- Lanuti M. Induction chemoradiotherapy for unresectable thymic tumors. Mediastinum 2017;1:13. [Crossref]
- Huang J, Detterbeck FC, Wang Z, et al. Standard outcome measures for thymic malignancies. J Thorac Oncol 2010;5:2017-23. [Crossref] [PubMed]
- Hamaji M, Ali SO, Burt BM. A meta-analysis of induction therapy for advanced thymic epithelial tumors. Ann Thorac Surg 2015;99:1848-56. [Crossref] [PubMed]
- Huang J, Rizk NP, Travis WD, et al. Feasibility of multimodality therapy including extended resections in stage IVA thymoma. J Thorac Cardiovasc Surg 2007;134:1477-83; discussion 1483-4. [Crossref] [PubMed]
- Park S, Ahn MJ, Ahn JS, et al. A prospective phase II trial of induction chemotherapy with docetaxel/cisplatin for Masaoka stage III/IV thymic epithelial tumors. J Thorac Oncol 2013;8:959-66. [Crossref] [PubMed]
- Rea F, Marulli G, Di Chiara F, et al. Multidisciplinary approach for advanced stage thymic tumors: long-term outcome. Lung Cancer 2011;72:68-72. [Crossref] [PubMed]
- Bierle DM, Raslau D, Regan DW, et al. Preoperative Evaluation Before Noncardiac Surgery. Mayo Clin Proc 2020;95:807-22. [Crossref] [PubMed]
- Goeddel LA, Grant MC. Preoperative Evaluation and Cardiac Risk Assessment in Vascular Surgery. Anesthesiol Clin 2022;40:575-85. [Crossref] [PubMed]
- Yamada Y, Yoshida S, Iwata T, et al. Risk factors for developing postthymectomy myasthenia gravis in thymoma patients. Ann Thorac Surg 2015;99:1013-9. [Crossref] [PubMed]
- Mineo TC, Mineo D, Onorati I, et al. New predictors of response to neoadjuvant chemotherapy and survival for invasive thymoma: a retrospective analysis. Ann Surg Oncol 2010;17:3022-9. [Crossref] [PubMed]
- Rena O, Mineo TC, Casadio C. Multimodal treatment for stage IVA thymoma: a proposable strategy. Lung Cancer 2012;76:89-92. [Crossref] [PubMed]
- Lucchi M, Melfi F, Dini P, et al. Neoadjuvant chemotherapy for stage III and IVA thymomas: a single-institution experience with a long follow-up. J Thorac Oncol 2006;1:308-13. [Crossref] [PubMed]
- Yamada Y, Yoshino I, Nakajima J, et al. Surgical Outcomes of Patients With Stage III Thymoma in the Japanese Nationwide Database. Ann Thorac Surg 2015;100:961-7. [Crossref] [PubMed]
- Riely GJ, Huang J. Induction therapy for locally advanced thymoma. J Thorac Oncol 2010;5:S323-6. [Crossref] [PubMed]
- Shintani Y, Funaki S, Ose N, et al. Surgical management of thymic epithelial tumors. Surg Today 2021;51:331-9. [Crossref] [PubMed]
- Funaki S, Shintani Y, Fukui E, et al. Surgical treatment strategies for invasive thymoma. J Thorac Dis 2020;12:7619-25. [Crossref] [PubMed]
- Fang W, Feng J, Ji C, et al. Minimally invasive thymectomy for locally advanced recurrent thymoma. J Vis Surg 2016;2:58. [Crossref] [PubMed]
- Hamaji M, Kojima F, Omasa M, et al. A meta-analysis of debulking surgery versus surgical biopsy for unresectable thymoma. Eur J Cardiothorac Surg 2015;47:602-7. [Crossref] [PubMed]
- Hishida T, Nomura S, Yano M, et al. Long-term outcome and prognostic factors of surgically treated thymic carcinoma: results of 306 cases from a Japanese Nationwide Database Study. Eur J Cardiothorac Surg 2016;49:835-41. [Crossref] [PubMed]
- Zhai Y, Hui Z, Gao Y, et al. Debulking Surgery Plus Radiation: Treatment Choice for Unresectable Stage III Thymic Carcinoma. Thorac Cardiovasc Surg 2020;68:440-5. [Crossref] [PubMed]
- Fan C, Ge H, Zhang S, et al. Impact of Definitive Radiotherapy and Surgical Debulking on Treatment Outcome and Prognosis for Locally Advanced Masaoka-Koga stage III Thymoma. Sci Rep 2020;10:1735. [Crossref] [PubMed]
- Mastromarino MG, Bacchin D, Aprile V, et al. Unradical Surgery for Locally-Advanced Thymoma: Is it time to evolve Perspectives? Lung Cancer 2023;180:107214. [Crossref] [PubMed]
- Kim ES, Putnam JB, Komaki R, et al. Phase II study of a multidisciplinary approach with induction chemotherapy, followed by surgical resection, radiation therapy, and consolidation chemotherapy for unresectable malignant thymomas: final report. Lung Cancer 2004;44:369-79. [Crossref] [PubMed]
- Park S, Park IK, Kim YT, et al. Comparison of Neoadjuvant Chemotherapy Followed by Surgery to Upfront Surgery for Thymic Malignancy. Ann Thorac Surg 2019;107:355-62. [Crossref] [PubMed]
- Kunitoh H, Tamura T, Shibata T, et al. A phase II trial of dose-dense chemotherapy, followed by surgical resection and/or thoracic radiotherapy, in locally advanced thymoma: report of a Japan Clinical Oncology Group trial (JCOG 9606). Br J Cancer 2010;103:6-11. [Crossref] [PubMed]
- Ahmad U, Huang J. Induction Therapy for Thymoma. Thorac Surg Clin 2016;26:325-32. [Crossref] [PubMed]
- Nakajo M, Takeda A, Katsuki A, et al. The efficacy of (18)F-FDG-PET-based radiomic and deep-learning features using a machine-learning approach to predict the pathological risk subtypes of thymic epithelial tumors. Br J Radiol 2022;95:20211050. [Crossref] [PubMed]
- Yang L, Cai W, Yang X, et al. Development of a deep learning model for classifying thymoma as Masaoka-Koga stage I or II via preoperative CT images. Ann Transl Med 2020;8:287. [Crossref] [PubMed]
- Kayi Cangir A, Orhan K, Kahya Y, et al. CT imaging-based machine learning model: a potential modality for predicting low-risk and high-risk groups of thymoma: "Impact of surgical modality choice". World J Surg Oncol 2021;19:147. [Crossref] [PubMed]
- Dapergola A, Gomatou G, Trontzas I, et al. Emerging therapies in thymic epithelial tumors Oncol Lett 2023;25:84. (Review). [Crossref] [PubMed]
- Tateo V, Manuzzi L, De Giglio A, et al. Immunobiology of Thymic Epithelial Tumors: Implications for Immunotherapy with Immune Checkpoint Inhibitors. Int J Mol Sci 2020;21:9056. [Crossref] [PubMed]
- Radovich M, Pickering CR, Felau I, et al. The Integrated Genomic Landscape of Thymic Epithelial Tumors. Cancer Cell 2018;33:244-258.e10. [Crossref] [PubMed]
- Tateo V, Manuzzi L, Parisi C, et al. An Overview on Molecular Characterization of Thymic Tumors: Old and New Targets for Clinical Advances. Pharmaceuticals (Basel) 2021;14:316. [Crossref] [PubMed]
- Petrini I, Meltzer PS, Kim IK, et al. A specific missense mutation in GTF2I occurs at high frequency in thymic epithelial tumors. Nat Genet 2014;46:844-9. [Crossref] [PubMed]
- Oberndorfer F, Müllauer L. Genomic alterations in thymoma-molecular pathogenesis? J Thorac Dis 2020;12:7536-44. [Crossref] [PubMed]
- Saito M, Fujiwara Y, Asao T, et al. The genomic and epigenomic landscape in thymic carcinoma. Carcinogenesis 2017;38:1084-91. [Crossref] [PubMed]
Cite this article as: Yamada Y, Hamaji M, Okada H, Takahagi A, Ajimizu H, Koyasu S, Sakamori Y, Aoyama A. Re-evaluation and operative indications after induction therapy for thymic epithelial tumors. Mediastinum 2024;8:43.