Diagnostic modalities in the mediastinum and the role of bronchoscopy in mediastinal assessment: a narrative review
Introduction
Mediastinum, as a thoracic compartment, encompasses critical structures including the heart, esophagus, trachea, main bronchi, thymus and major arteries and veins (1-3). A wide array of pathologies can occur in the mediastinum, most commonly lymphomas, lymphadenopathies, thymic masses, germ cell tumors and cystic lesions (4,5). Benign conditions such as sarcoidosis and atypical infections, including tuberculosis and fungal infections, also frequently affect the mediastinum.
Given the diverse range of diseases and anatomical structures within the mediastinum, numerous imaging modalities have been developed for its evaluation. These include chest X-ray (CXR), computed tomography (CT), magnetic resonance imaging (MRI), diffuse weighted imaging (DWI), and positron emission tomography (PET). While these imaging modalities have proven useful for evaluating mediastinal disease, they have notable limitations, such as exposure to contrast agents and radiation, and often fall short in providing a definitive diagnosis.
To overcome these limitations, invasive diagnostic modalities are essential. Among these, endobronchial ultrasound-transbronchial needle aspiration (EBUS-TBNA) has gained prominence for its efficacy in tissue sampling and lymph node biopsy. Historically, surgical exploration of the mediastinum with biopsy was the gold standard for diagnosis. However, the high diagnostic yield, accuracy, and safety profile of EBUS-TBNA has led to its rapid adoption and inclusion in guidelines as the first-line diagnostic modality.
This review explores the various imaging and invasive modalities for diagnosing mediastinal diseases, with a particular focus on bronchoscopic methods. We present this article in accordance with the Narrative Review reporting checklist (available at https://med.amegroups.com/article/view/10.21037/med-24-32/rc).
Methods
A literature search was performed via the PubMed database see Table 1. We included all types of articles and study design, including original research, meta-analyses, reviews, and abstracts.
Table 1
Items | Specification |
---|---|
Date of search | 06/01/2024 and 06/08/2024 |
Database searched | PubMed |
Search terms used | Examples of search terms used are: “Mediastinum Review”, “EBUS-TBNA Review”, “mediastinoscopy review”, “imaging modalities in the mediastinum review”, “radiographic techniques in the mediastinum review”, “EUS-FNA in the mediastinum review”, “confocal laser endomicroscopy in the mediastinum”, “optical coherence tomography in the mediastinum” |
Timeframe | 2009–2024 |
Inclusion and exclusion criteria | We included all types of articles and study design, including original research, meta-analyses, reviews, and abstracts. All articles used were in English. Studies performed prior to 2009 were excluded |
Selection process | All senior authors (Y.D., J.A.M.P., G.C.) consented to inclusion of each article in manuscript preparation. Only articles with complete consensus were included |
Radiographic techniques in the mediastinum
The International Thymic Malignancy Interest Group (ITIMG) classification was created to standardize localization of mediastinal masses on imaging and is widely accepted in clinical practice. ITIMG divides the mediastinum into three compartments: prevascular (anterior), visceral (middle), and paravertebral (posterior) (5). This classification system is used in various imaging modalities including CT, MRI, and fluorine-18-2-fluoro-2-deoxy-D-glucose-positron emission tomography (FDG-PET).
In 2020, the American College of Radiology (ACR) published appropriateness criteria recommending a step-wise approach to diagnosing a mediastinal mass. This approach begins with chest radiograph for general localization, followed by a CT chest for further characterization, FDG-PET is considered for additional metabolic information, leading to further diagnostic intervention if necessary. The ACR also recommends MRI of the chest to avoid unnecessary biopsy or surgery and to assist in planning for necessary procedures (6).
CXR
CXR is an imaging modality that utilizes X-rays that are absorbed by the structure of interest which causes attenuation of each ray and then exposes a film with different pixel values depending on that attenuation (see Figures 1,2). This results in a two-dimensional representation of a three-dimensional anatomical structure (7). Due to its simplicity and inexpensive nature, it is the most common initial modality used globally (7). This modality is recommended by ACR as the initial modality to localize a mediastinal mass and narrow the differential (6). Limitations of CXR include assessing smaller structures such as lymph nodes, further characterization of lesions is limited due to the composite attenuation of each X-ray beam, and image quality is often affected by patient positioning, degree of inspiration, medical devices, and body habitus (7).
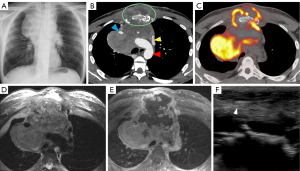
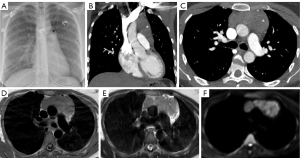
CT
CT imaging is the key modality for imaging the mediastinum because it is effective at localizing both malignant and benign disease in the mediastinum, is readily available, and can be performed rapidly. CT allows for increased resolution leading to more accurate assessment of location, size, presence of fat or calcification, attenuation, and relationship/involvement of surrounding and nearby organs (5,8). CT imaging uses a series of X-rays rotated around the patient, producing computer-generated additive cross-sectional images. This technique eliminates the superimposition of structures seen in CXR, removing noise and improving resolution (9). This increased resolution can be used to further characterize lymphomas, thymic neoplasms, and thyroid tumors (Figures 1-4) (4). There are several limitations to CT imaging of the mediastinum. CT imaging is less sensitive and specific than tissue diagnosis from more invasive techniques (10); one study showed CT was inferior to invasive techniques in both sensitivity and specificity in diagnosing small cell lung cancer (10). It is also often difficult to differentiate between solid and cystic lesions on CT especially if the cyst contains proteinaceous material or hemorrhage with high attenuation (11). CT also exposes the patient to higher radiation levels and is not suitable for patients that have allergies to iodinated contrast (6).
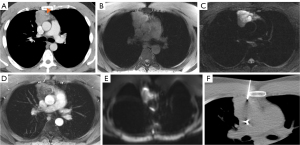
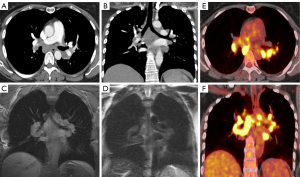
MRI
While MRI is not the initial diagnostic modality of choice in the mediastinum, it allows for further tissue characterization of mediastinal masses beyond that of CT (6). MRI has greater soft tissue resolution than CT, which is uniquely useful in revealing characteristics of mediastinal tumors (Figures 1-3). These features include cystic changes, hemorrhage, fat, fibrous capsules, and septa (12). MRI is also superior to CT in detection of mass invasion across tissue planes (6).
The key to these advantages is utilizing T1 and T2 weighted imaging available with MRI. T1 shows high signal intensity when imaging hemorrhage while T2 shows high signal intensity with cystic changes and myxoid tissue. Both are superior to CT when imaging fibrous capsules or septa in thymic tumors (12). Limitations of MRI include cost, patient claustrophobia, availability, lack of definitive diagnosis, and length of time needed to complete the exam.
DWI
DWI is a technique that generates signal contrast using principles of Brownian motion (i.e., motion of water molecules). A magnetic field gradient is produced by the MRI machine and a sensor measures the movement of random water molecules in response. This signal is then quantified into apparent diffusion coefficient (ADC) maps. Malignancies have been shown to have lower ADC values than surrounding tissue. These ADC values rise after treatment (13). A meta-analysis done by Santos et al. evaluated the role of DWI in differentiating mediastinal lymph nodes between benign and malignant, which showed a pooled sensitivity of 92% and specificity of 93% (14). Limitations of DWI include susceptibility to motion and arterial pulse artifact, nearby implants that can degrade study quality, and overlapping ADC values between malignant and benign lymph nodes (15).
Functional imaging
FDG-PET
FDG PET/CT is a functional imaging modality that utilizes radiotracers to measure active metabolic processes in the body (Figures 1,3,4). The role of FDG-PET in the mediastinum is primarily detection, staging, and surveillance of lymphadenopathy (6). In the case of malignancy or infection, it can also be useful in assessing response to treatment. FDG-PET has been shown to be more useful in excluding malignancy, while a positive FDG-PET is less helpful, as both benign and malignant processes can be PET avid (Figure 4) (6). This was illustrated by Tatci et al. who evaluated the ability of FDG PET/CT to differentiate malignant vs. benign masses; FDG PET/CT had a high sensitivity of 90%, but a low specificity at 55.16% (16).
Invasive techniques
The limited diagnostic capability of imaging modalities necessitates the use of invasive techniques to obtain tissue samples for pathological testing. This section summarizes some of the most common invasive techniques for obtaining tissue such as image-guided percutaneous core needle biopsy (CNB), endoscopic ultrasound (EUS), mediastinoscopy, and video-assisted thoracoscopy surgery (VATS). Mediastinoscopy is an open surgical approach, while EUS and VATS are considered minimally invasive surgeries.
Image-guided percutaneous CNB
Image guided CNB utilizes different imaging techniques (CT, CT-fluoroscopy, cone beam CT, ultrasonography, or MRI) to guide a needle for biopsy of a mediastinal lesion (Figure 3). This technique is minimally invasive and has less complications than the surgical procedures mentioned below (17). The diagnostic accuracy of CNB is variable across studies as there are different definitions of diagnostic accuracy. In one meta-analysis that pooled data from eighteen studies found the diagnostic accuracy was 92% while the total complication rate was 13%, and major complication rate was 2% (17). Major complications considered were pneumothorax requiring intervention, hemothorax, hemoptysis requiring intense care unit admission, air embolism, needles seeding of cancer, and death. The primary limitation of CNB is the inaccessibility of the target via percutaneous approach due to safety concerns arising from crowded anatomical space. Additional limitations include patient positioning challenges, navigation guidance relying on two-dimensional images, and patient allergies to iodinated contrast in the case of CT-guided procedures.
Endoscopic ultrasound-fine needle aspiration (EUS-FNA)
EUS-FNA is a minimally invasive technique that utilizes a high frequency ultrasound transducer incorporated into the tip of the endoscope to locate biopsy targets in the mediastinum with subsequent biopsy with a needle passing through the endoscope’s working channel. EUS refers to the introduction of the endoscope into the esophagus and thus is specifically suited for diagnosis of the middle and posterior mediastinum over an airway-centered approach, as the esophagus is located posteriorly to the trachea. Lymph node stations accessible with EUS include stations 1, 2L, 2R, 3P, 4L, 5–9, and 10L. Of these accessible stations, 5, 6, 8, and 9 are usually inaccessible to endobronchial ultrasound (EBUS) (18). EUS has been shown to be effective at diagnosing mediastinal disease in the locations it can access. In lung cancer, a meta-analysis analyzed 11 studies (n=313) and the pooled diagnostic yield was 90% (19). In diagnosing lymphadenopathy, other observational studies have shown a sensitivity of 88–95.3% and specificity of 96–100% (19). Other applications of EUS include pericardial effusion drainage, biopsy of pericardial masses, diagnosis of sarcoidosis, lymphoma, infections, and para-esophageal abscess drainage (19). There is a similar technique called endoscopic ultrasound with bronchoscope-guided fine-needle aspiration (EUS-B-FNA) where instead of using an endoscope, the EBUS bronchoscope is used sequentially to perform airway exam and then esophageal exam (20). Limitations of both EUS-FNA and EUS-B-FNA include, technical skills of the operator, expense, availability of endoscopy suite, constrained access to the anterior mediastinum, and narrow field of view (19).
VATS
VATS is a minimally invasive surgical procedure which involves small incisions in the chest wall and insertion of a thoracoscope to access the thoracic cavity. The goal of this approach is to minimize trauma to surrounding tissues. Similar to mediastinoscopy, it has been used for mediastinal tumor removal and lymph node biopsy allowing for staging. As VATS is a minimally invasive technique, it offers many advantages over open surgical procedures such as reduced post-operative pain, shorter hospital stays, and quicker recovery time (21). Despite these advantages, VATS carries significant risks such as hemorrhage, air embolism, pneumothorax, pleural effusion, wound infection, and postoperative pulmonary edema (21).
Mediastinoscopy
Mediastinoscopy is a surgical procedure which requires a small incision just above the manubrium and utilizes a mediastinoscope to examine the mediastinum, obtain tissue samples, remove tumors, and perform lymph node biopsy and staging. Most commonly, it has been utilized for diagnosing, treating, and staging non-small cell lung carcinoma (22). A study performed by Diebels et al. evaluated the utility of mediastinoscopy in mediastinal lymph node staging for non-small cell lung cancer (NSCLC). The study reported that mediastinoscopy had 81.8% sensitivity, 100% specificity, and 94.1% diagnostic accuracy (23). Due to the 100% specificity, mediastinoscopy was historically considered the gold standard for diagnosing the mediastinum especially for staging; however, it does carry significant risks similar to VATs such as hemorrhage, air embolism, injury to surrounding structures such as the recurrent laryngeal nerve, wound infection, and postoperative pulmonary edema (22). These risks result in morbidity rate of between 1.5% and 3%, and an overall mortality rate of 0.09% (22). Mediastinoscopy is best served for anterior mediastinal structures; accessing posterior mediastinum structures with mediastinoscopy is difficult.
Video-assisted mediastinal lymphadenectomy (VAMLA) and transcervical extended mediastinal lymphadenectomy (TEMLA)
Two surgical techniques were developed to supplement VATS in order to systematically stage the lymph nodes in the mediastinum and work by systematically removing all mediastinal lymph nodes along with the associated adipose tissue. VAMLA is achieved entirely through video assistance via insertion of a mediastinoscope through a small incision allowing for visualization and removal of most lymph nodes in the mediastinum. TEMLA is an open procedure where a 5–8 cm collar incision is made in the neck and then a Cooper retractor is used to lift the sternum allowing for visualization and complete dissection of all mediastinal lymph nodes. The main difference between the two techniques is that TEMLA has a higher negative predictive value (98.7%) because more lymph node stations are accessible (prevascular, paraaortic, subaortic and paraesophageal) but also has a higher rate of morbidity and mortality (24). Some studies suggest that these techniques have a high accuracy (96% and 98%) and a negative predictive value of 97–99% due to their increased sensitivity for micro metastases (25). Complications of VAMLA/TEMLA are similar to mediastinoscopy (mainly damage to surrounding structures, pneumothorax, pleural effusions, hemorrhage, and infection) and happen in less than 5% of patients. Data on the use of VAMLA and TEMLA has been mixed, and thus the routine use of these techniques is controversial. The latest European Society of Thoracic Surgery (ESTS) guidelines [2014] evaluated these two techniques and determined the data was scarce and thus did not recommend their use (26).
Advanced bronchoscopic imaging techniques
EBUS
The main role of EBUS in diagnosing the mediastinum is the real-time imaging of lymph nodes. There are three modes available with current EBUS technology to image lymph nodes; gray scale (B-mode), elastography, and blood flow Doppler (27). The most common and accepted mode is B-mode in combination with transbronchial needle aspiration (TBNA) (27). This section breaks down the utilization of these different techniques and the evidence supporting them.
Gray scale (B-mode) with EBUS-TBNA
Differentiating malignant and benign lymph node targets with B-mode imaging has both qualitative and quantitative methods. Qualitative methods include shape, size, echogenicity, margin, absence or presence of central hilar structure, nodal conglomeration, presence of coagulation necrosis sign, and presence of calcification (Figure 5) (27). The quantitative method uses gray scale texture to measure echogenicity the details of which are beyond the scope of this review (27).
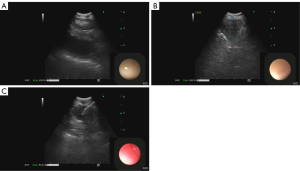
EBUS-TBNA involves bronchoscopic guidance of an ultrasound probe with adjacent working channel to different lymph node stations through the airways. The operator uses real-time gray scale ultrasound imaging to confirm the target. A fine needle is then used to make a transbronchial puncture into the lesion with subsequent aspiration of sample (Figures 5,6) (28). The decision of which lymph node to sample is based on size and morphology of the lymph node target. Lymph node locations accessible by EBUS are 2R, 2L, 3P, 4R, 4L and 7 as well as 10R, 10L, 11R, and 11L (28). In addition to lymph node targets, any other mediastinal pathology accessible from the bronchus, such as a mass, that can be identified on ultrasound can also be targeted. The advantages of this technique include its minimally invasive nature, does not necessitate general anesthesia, cost of procedure, does not require hospitalization, lower risk of complications such as pneumothorax compared to CT-guided biopsy, ability to target lymph nodes not accessible to other invasive techniques, decreased time to diagnosis, real-time imaging, ability to sample multiple lymph nodes and lesions in the same procedure, and high diagnostic yield.
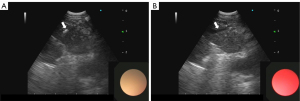
Many mediastinal diseases can be diagnosed by EBUS-TBNA. Malignant diseases include intrathoracic cancers (i.e., lung, thyroid, thymic, esophageal, lymphomas) as well as metastases from intrathoracic or extra-thoracic malignancies (29). Infectious causes of mediastinal lymphadenopathy diagnosed by EBUS-TBNA include Mycobacterium, fungi (Coccidioides, Histoplasma), other bacteria (F. tularensis, B. anthracis), and viruses (human immunodeficiency virus, Epstein-Barr virus) (29). Inflammatory causes include sarcoidosis, rheumatoid arthritis, and hypersensitivity pneumonitis (29).
EBUS-TBNA has now become standard of care for diagnosing and staging lung cancer, diagnosing and staging other mediastinal malignancies, and diagnosing infectious and benign mediastinal diseases. The American College of Chest Physicians (CHEST) recommends EBUS-TBNA as the initial diagnostic modality in mediastinal lung cancer staging, lymphadenopathy, sarcoidosis, tuberculosis, and lymphoma (30). The modality was recommended because of its high diagnostic yield and its minimally invasive safety profile.
In many studies prior to 2016, mediastinal staging of lung cancer with EBUS-TBNA had an overall diagnostic yield of 89% and negative predictive value of 91% (30). This high diagnostic yield led to a recommendation of using EBUS-TBNA as the initial mediastinal staging modality of choice over surgical staging. This was further supported by a more recent 2020 meta-analysis Kuijvenhoven et al. which analyzed 14 additional studies and reported a diagnostic yield of 89%, a sensitivity of 91%, with EBUS associated complications of only 5.4% (31).
For sarcoidosis, TBNA can be useful in providing histologic confirmation of non-caseating granulomatous inflammation. The 2016 CHEST guidelines reference the Agarwal et al. meta-analysis which included 15 studies which reported a diagnostic yield for sarcoidosis of 54–93% with a pooled diagnostic yield of 79% [95% confidence interval (CI): 71–86%] (32). Further analysis of 10 additional studies lead to a pooled diagnostic yield of 78.2% (95% CI: 75.6–80.4%) (32). The high diagnostic yield led to the CHEST recommendation of EBUS-TBNA as the initial diagnostic modality in suspected sarcoidosis in patients who have mediastinal or hilar lymphadenopathy. A more recent meta-analysis from Trisolini et al. reported a diagnostic yield of 79% (33).
For tuberculosis, the 2016 CHEST guidelines analyzed three studies. The diagnosis of tuberculosis via lymph node sampling was made by the presence of acid-fast bacilli smears on aspirate, or presence of necrotizing granuloma with positive tuberculosis skin test and right clinical context. The first two studies Garcia-Olivé et al. and Cetinkaya et al. had a high diagnostic yield of 80% and 79% respectively (34,35). The third study, Madan et al., showed a diagnostic yield of 84.8% (36). Based on these data, the CHEST guidelines recommended EBUS-TBNA as the initial diagnostic modality in suspected tuberculosis in patients that require lymph node sampling.
For lymphoma, the 2016 CHEST guidelines mention five studies with a total of 212 patients that evaluated the diagnostic yield of EBUS-TBNA. The two studies showing the highest diagnostic yield reported 91% and 89%; while the study showing the lowest diagnostic yield reported 38% (37) with an average diagnostic accuracy of 68.7% when all combined (30). The variability between the reported diagnostic yield between those studies was explained by differing definitions of diagnostic yield. Despite this variability, the data was sufficient to support the recommendation of EBUS-TBNA as the initial diagnostic modality (30).
Limitations of EBUS-TBNA
For mediastinal staging in lung cancer, EBUS is not able to stage the entire mediastinum. EBUS is limited to the anterosuperior mediastinum in areas accessible from larger bronchi. It is also technically difficult to access some anatomic areas such as the superior lung lobes due to the steep angle required for access. This limitation has been significantly decreased with the development of navigational bronchoscopy, but this topic is beyond the scope of this review. Effective EBUS requires a program including specially trained support staff, the proceduralist, and access to a pathology program that has the appropriate expertise. This can limit access to EBUS from hospitals that cannot field the cost (28).
EBUS elastography
Ultrasound elastography indirectly measures the stiffness and compressibility of lymph node tissue by assessing the speed of compression or shear waves penetrating the lymph node tissue and translating that into a color scale (Figure 7), allowing the operator to determine the stiffness of the lymph node (38). Malignant lymph node tissue has been shown in many studies to be stiffer than benign lymph node tissue (39,40).
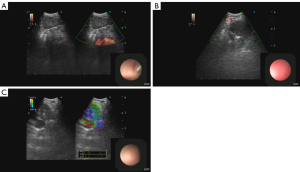
The best evidence suggesting diagnostic value of using elastography to differentiate between benign and malignant lymph nodes was a meta-analysis performed by Wu et al. (41). This study incorporated 17 studies and found a pooled sensitivity of 90% (95% CI: 0.84–0.94), and a specificity of 78% (95% CI: 0.74–0.81) for diagnosing mediastinal and hilar lymph nodes. These data led the authors of this study to recommend elastography as an adjunct to EBUS-TBNA to improve diagnostic accuracy. Of note, the studies analyzed in this meta-analysis were heterogenous and all elastography methods were included (quantitative, semi-quantitative, and qualitative). Thus, while these data are promising, the need for validating randomized trials as well as standardized elasticity measurement protocols remains.
Limitations of elastography
There are several limitations to elastography. False positives have been shown in benign diseases that cause increased stiffness such as sarcoidosis, pneumoconiosis, and tuberculosis as elastography cannot differentiate between stiff fibrotic lymph nodes and stiff malignant lymph nodes (42). False negatives have also been documented as necrosis, hemorrhage, or liquification of malignant lymph nodes also appear less stiff and thus may be incorrectly characterized as benign (42). Finally, there is a lack of standardization of elastography protocols; thus, further studies validating best practice protocols are still needed for wide-spread adoption.
Doppler imaging via EBUS
EBUS with flow Doppler takes advantage of the “Doppler effect” in physics where mass (red blood cells) moving toward or away from the detector (probe) changes the frequency of signal received (Figure 7). If the frequency detected increases, the flow is moving toward the probe and if the frequency decreases, away from the probe (43).
There are several qualitative methods for differentiating benign from malignant lymph nodes on color Doppler. One method described by Wang et al. classifies lymph nodes into three flow pattern distributions avascular, hilar vascular, and non-hilar vascular (44). Based on previous data showing that metastatic lymph nodes tend to have vascular supply but destroyed central hilar vasculature, they diagnosed malignant lymph nodes in the non-hilar vascular category. Using this classification system, the study cited a diagnostic accuracy of 79.69% (44). Another method described by Nakajima et al. (45) combined a similar flow pattern distribution with a graded vascular image pattern system where Grades 0 and I were benign and Grades II and III were malignant (45). The grades were determined by the number of blood vessels and the signal’s strength. Additionally, presence of positive bronchial artery (BA) inflow sign (blue signal towards a lymph node) was considered malignant regardless of grade. This system yielded a similar diagnostic accuracy of 78.0% (45). This grading system was further supported by a single-center prospective cohort trial in 2015 performed by Nosotti et al. who reported a diagnostic accuracy of 81% (46). No studies of quantitative methods of blood flow Doppler were found in our literature review. This diagnostic technique shows promise; however, more randomized studies as well as the development of quantitative techniques should be performed.
The limitation of Doppler flow imaging is no large studies have been performed to validate the Nakajima vascular pattern and because of the qualitative nature of the classification system, there is inherent subjectivity leading to inter-operator variability (46). Despite this limitation, this technology is already broadly integrated as an adjunct to EBUS-TBNA due to its precise identification of blood flow. The use of color Doppler to explicitly avoid vascular structures for needle sampling is widely used and enhances the safety profile of EBUS-TBNA.
Real time imaging and rapid on-site evaluation (ROSE)
ROSE is the process where a cytopathologist is present adjacent to the bronchoscopy suite and can provide real-time feedback on quality and quantity of the biopsy sample and sometimes provide an initial diagnosis (Figure 8). ROSE allows the cytopathologist to identify blood, necrosis, bronchial epithelial cells, lymphocytes, malignant cells, and atypical cells and relay that feedback to the operator (47). Chen et al. performed a meta-analysis, the pooled diagnostic yield of 18 studies showed improvement of 14% (95% CI: 0.09–0.18) when ROSE was used (47). There are conflicting studies on whether ROSE improves diagnostic yield. For instance, the Sehgal et al. meta-analysis analyzed five studies and showed no difference in diagnostic yield with the addition of ROSE [risk difference 0.04 (95% CI: –0.01 to 0.09)] (48). Despite the disagreement on diagnostic yield, most studies performed showed data supporting that ROSE decreases the number of needle passes; Chen et al. showed (−0.99, 95% CI: −1.89 to −0.09) and Sehgal et al. showed a mean difference of –1.1 (95% CI: –2.2 to –0.005; P<0.001) (47,48). However, neither study saw a difference in complication rate. While ROSE does not significantly alter diagnostic yield, it does reduce the number of needle passes needed.
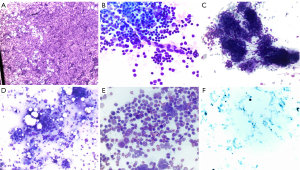
Comparative analysis
EBUS and traditional imaging/surgical modalities
Many guidelines recommend EBUS-TBNA as a first line modality for mediastinal diagnosis. These guidelines were supported by comparison studies between EBUS-TBNA, CT-guided biopsy, and mediastinoscopy.
EBUS-TBNA and FDG-PET
Several studies have shown EBUS-TBNA to have higher diagnostic accuracy than PET. One study showed that EBUS-TBNA has a higher accuracy (87.5% vs. 79.2%), positive predictive value, and specificity as PET (49). More recently, the SEISMIC study found that systematic endoscopic mediastinal staging was more accurate with addition of EBUS-TBNA than FDG-PET alone in diagnosing NSCLC (50). While the diagnostic accuracy has been shown to be higher in EBUS-TBNA, the two techniques are both involved in the staging of mediastinum. According to the ESTS guidelines [2014], suspicion for nodal disease on CT chest or FDG-PET prompts mediastinal staging with EBUS/EUS (26).
EBUS-TBNAs and CT-guided biopsy
Many studies have compared diagnostic accuracy and complication rate between EBUS-TBNA and CT-guided biopsy. A study by Uchimura et al. explored EBUS with elastography analysis of lymph nodes that were considered negative by CT. The results showed EBUS with elastography using an SAR cutoff had a sensitivity of 88%, specificity 80.2%, and diagnostic accuracy of 83.9% (51). This study suggested that even in radiographically negative lymph nodes, EBUS with elastography still predicted malignant lymph nodes with high diagnostic accuracy (51). While EBUS with elastography can catch some radiographically negative lymph nodes, when directly comparing the two techniques, the diagnostic yield has been shown to be similar. A meta-analysis by Fu et al. analyzed nine studies and found that both diagnostic yield [odds ratio (OR): 0.23; P<0.001] and diagnostic accuracy (OR: 0.43; P=0.002) was higher with CT guided biopsy than with EBUS-TBNA with similar rates of procedural success. However, the complication rate of CT guided biopsy was substantially higher (OR: 7.27; P<0.001) (52). The authors also noted that there was large heterogenicity in the way studies defined diagnostic yield. Sub-group analysis performed showed that CT guided biopsy was particularly superior in diagnostic yield when the lesions were small or close to the pleura. When reviewing the data, both EBUS-TBNA and CT-guided biopsy have utility in diagnosing the mediastinum, but CT-guided biopsy does have a higher complication rate.
EBUS-TBNA and mediastinoscopy
Historically, mediastinoscopy has been considered the gold standard for invasive diagnosis of mediastinal disease. This changed around 2016 with data supporting the use of EBUS-TBNA as the first-line diagnostic modality due to being more cost-effective, lower complication rate, and a similar to better (in some studies) diagnostic yield (53). Many studies have shown that EBUS-TBNA is equivalent to or better than mediastinoscopy in terms of diagnostic yield in lymph node staging of NSCLC. Um et al. showed accuracy of 92.9%, sensitivity of 88%, and specificity 100% compared to mediastinoscopy which showed 89%, 81.3%, and 100% respectively (54). Yasufuku et al. showed sensitivity, specificity, and diagnostic accuracy for EBUS- TBNA was 81%, 100%, and 93% and for mediastinoscopy 79%, 100%, and 93% respectively (55). No complications were seen in EBUS patients and 2.6% had complications in mediastinoscopy (55). A more recent 2020 meta-analysis performed by Figueiredo et al. analyzed five studies and found that no statistically significant differences were observed in sensitivity, specificity, positive predictive value, or negative predictive value between the two modalities, but they did not assess diagnostic accuracy (56).
Even though the data does support EBUS-TBNA as a first-line modality over mediastinoscopy due to similar diagnostic yield with lower complication rate, there remains a role for mediastinoscopy in diagnosis of the mediastinum in select patients that are EBUS-TBNA negative or have no pulmonary manifestations of disease. For example, Zhu et al. found that in patients with lymphadenopathy but without pulmonary abnormalities, mediastinoscopy had a much higher diagnostic accuracy than EBUS-TBNA (96% vs. 62%) (57).
Synergistic use of EBUS with other modalities
While EBUS-TBNA has revolutionized the diagnosis of the mediastinum, its ability to achieve a definitive diagnosis can be further augmented by combining it with elastography, EUS-FNA, and mediastinoscopy.
EBUS-TBNA with EUS-FNA
There are several advantages to combining these two modalities including ability to access inferior mediastinal lymph nodes, reaching para-esophageal masses not normally accessible to EBUS-TBNA, and increased diagnostic yield (58). Further, if EBUS-TBNA is combined with EUS-B-FNA (inserting the EBUS scope into the esophagus to perform the EUS exam), then there are many other advantages such as decreased cost, lower doses of sedatives, less incidence of oxygen desaturation in low reserve patients, operator satisfaction, shorter procedural time, ability to be performed by a single operator, and ability to perform procedure in a single setting (58). A meta-analysis performed by Hong et al. analyzed studies with combined EBUS-TBNA and EUS-B-FNA in diagnosing and staging lung cancer and sarcoidosis. In lung cancer, 10 prospective studies involving greater than 1,000 patients, all showed a significant increase in both diagnostic yield and sensitivity with the combination (58). In sarcoidosis, Hong et al. reported there were no prospective head-to-head studies comparing combination EBUS-TBNA/EUS-B-FNA to EBUS-TBNA alone, but there were two studies that reported a diagnostic yield of 88% and 66% respectively for EBUS-TBNA alone (59,60). This was then compared to a third study which reported, for the combination modality, a diagnostic yield of 92.16% (61). This suggests that the combination modality produced a higher yield (58).
Another meta-analysis performed by Shen et al. analyzed 10 studies that compared EBUS-TBNA with EUS-FNA, and six studies that compared EBUS-TBNA with EUS-B-FNA all in diagnosing general mediastinal disease. They concluded that diagnostic accuracy was slightly better in the EBUS-TBNA/EUS-FNA combination (62). In summary, the combination of EBUS-TBNA with either EUS-B-FNA or EUS-FNA can significantly improve the diagnostic yield in appropriate cases.
EBUS-TBNA with mediastinoscopy
While current guidelines recommend EBUS-TBNA for first line diagnosis of lung cancer and lymph node staging, there is significant data supporting the use of video-assisted mediastinoscopy (VAM) in patients with EBUS-TBNA negative lymph nodes. A meta-analysis performed by Sanz-Santos et al. analyzed 28 studies of patients with EBUS-TBNA negative lymph nodes. Of those patients, 2,472 came from studies without confirmatory VAM and 2,721 came from studies with confirmatory VAM. The meta-analysis reported that sensitivity and negative predictive value were improved in patients with confirmatory VAM (66.9% increased to 96.7% and 79.2% increased to 91.8% respectively) (63). Despite these significant improvements, the number needed to treat (NNT) was high (23.8). The authors concluded confirmatory VAM after negative EBUS-TBNA reduced the rate of unforeseen N2/N3 disease, but the NNT was high (63). With the high NNT, only certain patient populations should be offered confirmatory VAM (63). While Sanz-Santos et al. did not comment on which patient populations this should be, many different studies and current guidelines suggest those patients with negative EBUS-TBNA lymph nodes with high pre-test probability of malignancy should receive confirmatory VAM.
EBUS-TBNA with forceps and cryobiopsy
Staging and diagnosis of the mediastinum with EBUS continues to improve as new tools allow for larger tissue samples. Forceps biopsy has been shown to increase diagnostic yield especially in sarcoidosis or lymphoma due to the large tissue sample acquired (64). Transbronchial biopsy with the 1.1 mm cryoprobe has also been shown to increase diagnostic yield with a larger tissue sample and the added benefit of preserving tissue architecture. This has been particularly useful in diagnosing interstitial lung disease (64). Several studies have shown augmented diagnostic yield with these tools over EBUS-TBNA. Two RCTs showed an augmented diagnostic yield with cryobiopsy (93% from 81% and 91.6% from 85.7%) (64,65). Additionally, a study showed cryobiopsy yielded tissue more qualified for lung cancer molecular testing then forceps (64). A meta-analysis performed by Mathew et al. (N=844), showed the cryobiopsy augmented EBUS-TBNA from diagnostic yield of 81% to 91% with no significant increases in complications (66). The development of advanced tools such as the cryo probe, continues to increase the efficacy of EBUS as a diagnostic technique in the mediastinum.
Future directions in mediastinal diagnosis with bronchoscopy
The field of bronchoscopy is continuously advancing, and new techniques and technologies are showing promise in advancing the field of mediastinal diagnosis. Some of these new techniques/technologies include confocal laser endomicroscopy (CLE), optical coherence tomography (OCT), and artificial intelligence (AI).
CLE
CLE is a novel technique that is minimally invasive and provides real-time, microscopic analysis of tissue structure (67). This procedure involves advancing a flexible mini-probe (pCLE) using transbronchial or trans-esophageal procedures or transbronchial/trans-esophageal biopsy needles (nCLE). Once located at the specimen of interest, a low power laser is shined on the specimen and the reflected light travels back up a pin hole to the detector. Out of focus light is thus blocked by the pin hole allowing for microscopic imaging (67). This technology has been useful in diagnosing lung disease, but it can equally be applied to mediastinum. There have been preliminary observational studies that have shown promise in CLE diagnosis of mediastinal lymph nodes. Benias et al. performed an observational study during EUS procedures and found that clusters of dark pleomorphic cells could be seen on nCLE and correlated well with histologic proven tumor cells (68). Wijmans et al. (69) also performed an observational study where they used three nCLE criteria (dark enlarged pleomorphic cells, dark clumps, and directional streaming) to detect malignancy. A study by Zuo et al. found that combining nCLE with EBUS resulted in a higher sensitivity, specificity, and positive predictive value, than either modality alone (70). While this technology has shown promise in preliminary studies in diagnosing malignancy in mediastinal lymph nodes, confirmatory larger randomized trials are needed before widespread adoption.
OCT
OCT is a near infra-red light-based technique that allows for high resolution images of target tissues. The OCT probe can be advanced through the working channel of the EBUS scope or regular bronchoscope allowing for real-time high-resolution images of lymph nodes of interest in the mediastinum (71). These images are produced by generating pull backs from distal to proximal, and then taking the resultant circumferential two-dimensional images and reconstructing them to three dimensional images (71). While there have been a few ex-vivo preliminary studies using OCT, we were unable to find any published in-vivo studies in our literature review. Hariri et al. developed OCT criteria in an ex-vivo study for differentiating solitary pulmonary nodules (SPN) and lung parenchyma (72). Shostak et al. later further observed characteristics that distinguished carcinoma from benign lymph node tissue in another ex-vivo study (73). Future studies are needed to be able to distinguish lymph node tissue from bronchial or lung parenchyma, but this technology shows promise in enhancing future EBUS-TBNA procedures.
AI
With the widespread adoption of AI, there have been many preliminary studies applying AI to bronchoscopy. A subset of AI called “machine learning” is the process of building computer models that can learn and then make predictions from data without being explicitly programmed. The main application of AI in bronchoscopy has been using intraluminal bronchial images that has allowed the “deep learning” AI to identify bronchial segments with a high degree of accuracy. Additionally, EBUS images have been utilized to instruct the AI on how to recognize benign vs. malignant disease (74). Another subset of AI termed “artificial neural networks” or “convolutional neural networks (CNN)” uses sensory “neurons” as data inputs which then connect to a hidden layer of computer programming and then transferred to an output layer that renders the decision (74). These CNN models may be helpful for training future bronchoscopists since the CNN can provide real time bronchial anatomy feedback to the operator. Cold et al. studied bronchoscopy training between a group using the CNN model for real time feedback vs. written instructions. The group using the CNN model showed significantly higher performance in segment identification (75). These studies are small and preliminary and larger confirmatory trials are needed before widespread adoption.
Deep learning models also shows promise in expanding access to ROSE. Yan et al. compared diagnostic accuracy of a deep learning model to a cytopathologist during flexible bronchoscopy with transbronchial biopsy (76). Their model showed comparable to slightly inferior accuracy to the pathologists in both image identification as well as in cancer diagnosis (76). This capability could make ROSE more available to healthcare facilities that do not have access to ROSE pathologists (76). While this is an exciting potential application of AI, further confirmatory studies are needed before widespread adoption.
Limitations of the review
Our review has several limitations. This is a “narrative” review and thus the data presented is not comprehensive. Not all data presented comes from prospective randomized controlled trials and no analysis for bias was performed; thus, there may be inherent bias in the data presented. Studies presented had different definitions of diagnostic accuracy and diagnostic yield so comparison between these studies should be made with caution. Studies presented were performed in many different countries in heterogeneous patient populations, so comparison between patient populations should be made with caution.
Conclusions
Bronchoscopy with its versatility, recent advancement, and high diagnostic yields with relatively low complication rate has revolutionized invasive diagnosis of the mediastinum. In both benign and malignant diseases, bronchoscopy has given patients and providers unprecedented cost savings, decreased time to diagnosis, decreased complications, and has led to better health outcomes. Bronchoscopy has also been proven to have even better diagnostic yield and outcomes when combined with classic imaging and diagnostic modalities such as CT, PET, MRI, EUS, and surgical mediastinoscopy. Bronchoscopy continues to advance mediastinal diagnosis with innovative technologies such as elastography, CLE, OCT, and AI. In conclusion, bronchoscopy is and will continue to be an integral modality in invasive diagnosis of the mediastinum.
Acknowledgments
We thank Gina Mautz, a coordinator from the Interventional Pulmonary Department, for her administrative support and the Interventional Pulmonary Department at the University of California San Diego for the administrative support.
Funding: None.
Footnote
Reporting Checklist: The authors have completed the Narrative Review reporting checklist. Available at https://med.amegroups.com/article/view/10.21037/med-24-32/rc
Peer Review File: Available at https://med.amegroups.com/article/view/10.21037/med-24-32/prf
Conflicts of Interest: All authors have completed the ICMJE uniform disclosure form (available at https://med.amegroups.com/article/view/10.21037/med-24-32/coif). The authors have no conflicts of interest to declare.
Ethical Statement: The authors are accountable for all aspects of the work in ensuring that questions related to the accuracy or integrity of any part of the work are appropriately investigated and resolved. All procedures performed in this study were in accordance with the ethical standards of the institutional and/or national research committee(s) and with the Helsinki Declaration (as revised in 2013). Written informed consent was obtained from the patient for the publication of this study and accompanying images. A copy of the written consent is available for review by the editorial office of this journal.
Open Access Statement: This is an Open Access article distributed in accordance with the Creative Commons Attribution-NonCommercial-NoDerivs 4.0 International License (CC BY-NC-ND 4.0), which permits the non-commercial replication and distribution of the article with the strict proviso that no changes or edits are made and the original work is properly cited (including links to both the formal publication through the relevant DOI and the license). See: https://creativecommons.org/licenses/by-nc-nd/4.0/.
References
- Rizvi S, Wehrle CJ, Law MA. Anatomy, Thorax, Mediastinum Superior and Great Vessels. 2024.
- Stoddard N, Heil JR, Lowery DR. Anatomy, Thorax, Mediastinum. [Updated 2023 Jul 24]. Treasure Island (FL): StatPearls Publishing; 2024.
- Ghigna MR, Thomas de Montpreville V. Mediastinal tumours and pseudo-tumours: a comprehensive review with emphasis on multidisciplinary approach. Eur Respir Rev 2021;30:200309. [Crossref] [PubMed]
- Taka M, Kobayashi S, Mizutomi K, et al. Diagnostic approach for mediastinal masses with radiopathological correlation. Eur J Radiol 2023;162:110767. [Crossref] [PubMed]
- Ahuja J, Strange CD, Agrawal R, et al. Approach to Imaging of Mediastinal Masses. Diagnostics (Basel) 2023;13:3171. [Crossref] [PubMed]
- Expert Panel on Thoracic Imaging. ACR Appropriateness Criteria® Imaging of Mediastinal Masses. J Am Coll Radiol 2021;18:S37-51. [Crossref] [PubMed]
- Jones CM, Buchlak QD, Oakden-Rayner L, et al. Chest radiographs and machine learning - Past, present and future. J Med Imaging Radiat Oncol 2021;65:538-44. [Crossref] [PubMed]
- Iyer H, Anand A, Sryma PB, et al. Mediastinal lymphadenopathy: a practical approach. Expert Rev Respir Med 2021;15:1317-34. [Crossref] [PubMed]
- Patel PR, De Jesus O. CT Scan. StatPearls. Treasure Island (FL): StatPearls Publishing LLC.; 2024.
- Fréchet B, Kazakov J, Thiffault V, et al. Diagnostic Accuracy of Mediastinal Lymph Node Staging Techniques in the Preoperative Assessment of Nonsmall Cell Lung Cancer Patients. J Bronchology Interv Pulmonol 2018;25:17-24. [Crossref] [PubMed]
- Jo N, Shroff GS, Wu CC, et al. Imaging of the mediastinum: Mimics of malignancy. Semin Diagn Pathol 2022;39:92-8. [Crossref] [PubMed]
- Nakazono T, Yamaguchi K, Egashira R, et al. MRI Findings and Differential Diagnosis of Anterior Mediastinal Solid Tumors. Magn Reson Med Sci 2023;22:415-33. [Crossref] [PubMed]
- Baliyan V, Das CJ, Sharma R, et al. Diffusion weighted imaging: Technique and applications. World J Radiol 2016;8:785-98. [Crossref] [PubMed]
- Santos FS, Verma N, Watte G, et al. Diffusion-weighted magnetic resonance imaging for differentiating between benign and malignant thoracic lymph nodes: a meta-analysis. Radiol Bras 2021;54:225-31. [Crossref] [PubMed]
- Thuy TTM, Trang NTH, Vy TT, et al. Role of diffusion-weighted MRI in differentiation between benign and malignant anterior mediastinal masses. Front Oncol 2022;12:985735. [Crossref] [PubMed]
- Tatci E, Ozmen O, Dadali Y, et al. The role of FDG PET/CT in evaluation of mediastinal masses and neurogenic tumors of chest wall. Int J Clin Exp Med 2015;8:11146-52. [PubMed]
- Lee HN, Yun SJ, Kim JI, et al. Diagnostic outcome and safety of CT-guided core needle biopsy for mediastinal masses: a systematic review and meta-analysis. Eur Radiol 2020;30:588-99. [Crossref] [PubMed]
- Ko J, Hegde P. Added value of endoscopic ultrasound to endobronchial ultrasound in non-small cell lung cancer staging. AME Med J 2024;9:2. [Crossref]
- Okasha HH, El-Meligui A, Pawlak KM, et al. Practical approach to linear EUS examination of the mediastinum. Endosc Ultrasound 2021;10:406-13. [Crossref] [PubMed]
- Bugalho A, de Santis M, Slubowski A, et al. Trans-esophageal endobronchial ultrasound-guided needle aspiration (EUS-B-NA): A road map for the chest physician. Pulmonology 2017;24:32-41. [Crossref] [PubMed]
- Mehrotra M, D'Cruz JR, Bishop MA, et al. Video-Assisted Thoracoscopy. [Updated 2024 May 1]. Treasure Island (FL): StatPearls Publishing; 2024.
- McNally P, Arthur M. Mediastinoscopy. [Updated 2022 Sep 12]. StatPearls [Internet] Treasure Island (FL): StatPearls Publishing; 2022.
- Diebels I, Hendriks JMH, Van Meerbeeck JP, et al. Evaluation of mediastinoscopy in mediastinal lymph node staging for non-small-cell lung cancer. Interact Cardiovasc Thorac Surg 2021;32:270-5. [Crossref] [PubMed]
- Hartert M, Tripsky J, Huertgen M. Video-assisted mediastinoscopic lymphadenectomy (VAMLA) for staging & treatment of non-small cell lung cancer (NSCLC). Mediastinum 2020;4:3. [Crossref] [PubMed]
- Leiro-Fernández V, Fernández-Villar A. Mediastinal staging for non-small cell lung cancer. Transl Lung Cancer Res 2021;10:496-505. [Crossref] [PubMed]
- De Leyn P, Dooms C, Kuzdzal J, et al. Revised ESTS guidelines for preoperative mediastinal lymph node staging for non-small-cell lung cancer. Eur J Cardiothorac Surg 2014;45:787-98. [Crossref] [PubMed]
- Zhi X, Chen J, Xie F, et al. Diagnostic value of endobronchial ultrasound image features: A specialized review. Endosc Ultrasound 2021;10:3-18. [Crossref] [PubMed]
- Jalil BA, Yasufuku K, Khan AM. Uses, limitations, and complications of endobronchial ultrasound. Proc (Bayl Univ Med Cent) 2015;28:325-30. [Crossref] [PubMed]
- Scano V, Fois AG, Manca A, et al. Role of EBUS-TBNA in Non-Neoplastic Mediastinal Lymphadenopathy: Review of Literature. Diagnostics (Basel) 2022;12:512. [Crossref] [PubMed]
- Wahidi MM, Herth F, Yasufuku K, et al. Technical Aspects of Endobronchial Ultrasound-Guided Transbronchial Needle Aspiration: CHEST Guideline and Expert Panel Report. Chest 2016;149:816-35. [Crossref] [PubMed]
- Kuijvenhoven JC, Leoncini F, Crombag LC, et al. Endobronchial Ultrasound for the Diagnosis of Centrally Located Lung Tumors: A Systematic Review and Meta-Analysis. Respiration 2020;99:441-50. [Crossref] [PubMed]
- Agarwal R, Srinivasan A, Aggarwal AN, et al. Efficacy and safety of convex probe EBUS-TBNA in sarcoidosis: a systematic review and meta-analysis. Respir Med 2012;106:883-92. [Crossref] [PubMed]
- Trisolini R, Lazzari Agli L, Tinelli C, et al. Endobronchial ultrasound-guided transbronchial needle aspiration for diagnosis of sarcoidosis in clinically unselected study populations. Respirology 2015;20:226-34. [Crossref] [PubMed]
- Garcia-Olivé I, Valverde Forcada EX, Andreo García F, et al. Linear endobronchial ultrasound as the initial diagnostic tool in patients with indications of mediastinal disease. Arch Bronconeumol 2009;45:266-70. [Crossref] [PubMed]
- Cetinkaya E, Gunluoglu G, Ozgul A, et al. Value of real-time endobronchial ultrasound-guided transbronchial needle aspiration. Ann Thorac Med 2011;6:77-81. [Crossref] [PubMed]
- Madan K, Mohan A, Ayub II, et al. Initial experience with endobronchial ultrasound-guided transbronchial needle aspiration (EBUS-TBNA) from a tuberculosis endemic population. J Bronchology Interv Pulmonol 2014;21:208-14. [Crossref] [PubMed]
- Iqbal S, DePew ZS, Kurtin PJ, et al. Endobronchial ultrasound and lymphoproliferative disorders: a retrospective study. Ann Thorac Surg 2012;94:1830-4. [Crossref] [PubMed]
- Sigrist RMS, Liau J, Kaffas AE, et al. Ultrasound Elastography: Review of Techniques and Clinical Applications. Theranostics 2017;7:1303-29. [Crossref] [PubMed]
- Ying L, Hou Y, Zheng HM, et al. Real-time elastography for the differentiation of benign and malignant superficial lymph nodes: a meta-analysis. Eur J Radiol 2012;81:2576-84. [Crossref] [PubMed]
- Verhoeven RLJ, de Korte CL, van der Heijden EHFM. Optimal Endobronchial Ultrasound Strain Elastography Assessment Strategy: An Explorative Study. Respiration 2019;97:337-47. [Crossref] [PubMed]
- Wu J, Sun Y, Wang Y, et al. Diagnostic value of endobronchial ultrasound elastography for differentiating benign and malignant hilar and mediastinal lymph nodes: a systematic review and meta-analysis. Med Ultrason 2022;24:85-94. [Crossref] [PubMed]
- Huang J, Lu Y, Wang X, et al. Diagnostic value of endobronchial ultrasound elastography combined with rapid onsite cytological evaluation in endobronchial ultrasound-guided transbronchial needle aspiration. BMC Pulm Med 2021;21:423. [Crossref] [PubMed]
- Oglat AA, Matjafri MZ, Suardi N, et al. A Review of Medical Doppler Ultrasonography of Blood Flow in General and Especially in Common Carotid Artery. J Med Ultrasound 2018;26:3-13. [Crossref] [PubMed]
- Wang L, Wu W, Hu Y, et al. Sonographic Features of Endobronchial Ultrasonography Predict Intrathoracic Lymph Node Metastasis in Lung Cancer Patients. Ann Thorac Surg 2015;100:1203-9. [Crossref] [PubMed]
- Nakajima T, Anayama T, Shingyoji M, et al. Vascular image patterns of lymph nodes for the prediction of metastatic disease during EBUS-TBNA for mediastinal staging of lung cancer. J Thorac Oncol 2012;7:1009-14. [Crossref] [PubMed]
- Nosotti M, Palleschi A, Tosi D, et al. Color-Doppler sonography patterns in endobronchial ultrasound-guided transbronchial needle aspiration of mediastinal lymph-nodes. J Thorac Dis 2017;9:S376-80. [Crossref] [PubMed]
- Chen X, Wan B, Xu Y, et al. Efficacy of rapid on-site evaluation for diagnosing pulmonary lesions and mediastinal lymph nodes: a systematic review and meta-analysis. Transl Lung Cancer Res 2019;8:1029-44. [Crossref] [PubMed]
- Sehgal IS, Dhooria S, Aggarwal AN, et al. Impact of Rapid On-Site Cytological Evaluation (ROSE) on the Diagnostic Yield of Transbronchial Needle Aspiration During Mediastinal Lymph Node Sampling: Systematic Review and Meta-Analysis. Chest 2018;153:929-38. [Crossref] [PubMed]
- Al-Ibraheem A, Hirmas N, Fanti S, et al. Impact of (18)F-FDG PET/CT, CT and EBUS/TBNA on preoperative mediastinal nodal staging of NSCLC. BMC Med Imaging 2021;21:49. [Crossref] [PubMed]
- Steinfort DP, Kothari G, Wallace N, et al. Systematic endoscopic staging of mediastinum to guide radiotherapy planning in patients with locally advanced non-small-cell lung cancer (SEISMIC): an international, multicentre, single-arm, clinical trial. Lancet Respir Med 2024;12:467-75. [Crossref] [PubMed]
- Uchimura K, Yamasaki K, Sasada S, et al. Quantitative analysis of endobronchial ultrasound elastography in computed tomography-negative mediastinal and hilar lymph nodes. Thorac Cancer 2020;11:2590-9. [Crossref] [PubMed]
- Fu YF, Zhang JH, Wang T, et al. Endobronchial ultrasound-guided versus computed tomography-guided biopsy for peripheral pulmonary lesions: A meta-analysis. Clin Respir J 2021;15:3-10. [Crossref] [PubMed]
- Czarnecka-Kujawa K, Yasufuku K. The role of endobronchial ultrasound versus mediastinoscopy for non-small cell lung cancer. J Thorac Dis 2017;9:S83-97. [Crossref] [PubMed]
- Um SW, Kim HK, Jung SH, et al. Endobronchial ultrasound versus mediastinoscopy for mediastinal nodal staging of non-small-cell lung cancer. J Thorac Oncol 2015;10:331-7. [Crossref] [PubMed]
- Yasufuku K, Pierre A, Darling G, et al. A prospective controlled trial of endobronchial ultrasound-guided transbronchial needle aspiration compared with mediastinoscopy for mediastinal lymph node staging of lung cancer. J Thorac Cardiovasc Surg 2011;142:1393-400.e1. [Crossref] [PubMed]
- Figueiredo VR, Cardoso PFG, Jacomelli M, et al. EBUS-TBNA versus surgical mediastinoscopy for mediastinal lymph node staging in potentially operable non-small cell lung cancer: a systematic review and meta-analysis. J Bras Pneumol 2020;46:e20190221. [Crossref] [PubMed]
- Zhu F, Ma DC, Xu N, et al. Diagnostic Value of Video-assisted Mediastinoscopy and Endobronchial Ultrasound-guided Transbronchial Needle Aspiration for Mediastinal Lymphadenectasis without Pulmonary Abnormalities. Med Sci Monit 2017;23:3064-70. [Crossref] [PubMed]
- Hong G, Oki M. Transesophageal endoscopic ultrasound with bronchoscope-guided fine-needle aspiration for diagnostic and staging purposes: a narrative review. J Thorac Dis 2023;15:5088-98. [Crossref] [PubMed]
- Oki M, Saka H, Kitagawa C, et al. Transesophageal bronchoscopic ultrasound-guided fine needle aspiration for diagnosis of sarcoidosis. Respiration 2013;85:137-43. [Crossref] [PubMed]
- Crombag LMM, Mooij-Kalverda K, Szlubowski A, et al. EBUS versus EUS-B for diagnosing sarcoidosis: The International Sarcoidosis Assessment (ISA) randomized clinical trial. Respirology 2022;27:152-60. [Crossref] [PubMed]
- Filarecka A, Gnass M, Wojtacha J, et al. Usefulness of combined endobronchial and endoscopic ultrasound-guided needle aspiration in the diagnosis of sarcoidosis: a prospective multicenter trial. Pol Arch Intern Med 2020;130:582-8. [Crossref] [PubMed]
- Shen Y, Qin S, Jiang H. Endobronchial ultrasound-guided transbronchial needle aspiration combined with either endoscopic ultrasound-guided fine-needle aspiration or endoscopic ultrasound using the EBUS scope-guided fine-needle aspiration for diagnosing and staging mediastinal diseases: a systematic review and meta-analysis. Clinics (Sao Paulo) 2020;75:e1759. Erratum in: Clinics (Sao Paulo) 2020;75:e1759err. [Crossref] [PubMed]
- Sanz-Santos J, Almagro P, Malik K, et al. Confirmatory Mediastinoscopy after Negative Endobronchial Ultrasound-guided Transbronchial Needle Aspiration for Mediastinal Staging of Lung Cancer: Systematic Review and Meta-analysis. Ann Am Thorac Soc 2022;19:1581-90. [Crossref] [PubMed]
- Cheng TL, Huang ZS, Zhang J, et al. Comparison of cryobiopsy and forceps biopsy for the diagnosis of mediastinal lesions: A randomised clinical trial. Pulmonology 2024;30:466-74. [Crossref] [PubMed]
- Fan Y, Zhang AM, Wu XL, et al. Transbronchial needle aspiration combined with cryobiopsy in the diagnosis of mediastinal diseases: a multicentre, open-label, randomised trial. Lancet Respir Med 2023;11:256-64. [Crossref] [PubMed]
- Mathew R, Roy WE, Thomas ES, et al. Meta-analysis and systematic review of mediastinal cryobiopsy versus endobronchial ultrasound-transbronchial needle aspiration (EBUS-TBNA) in the diagnosis of intrathoracic adenopathy. J Thorac Dis 2024;16:4217-28. [Crossref] [PubMed]
- Tian S, Huang H, Zhang Y, et al. The role of confocal laser endomicroscopy in pulmonary medicine. Eur Respir Rev 2023;32:220185. [Crossref] [PubMed]
- Benias PC, D'Souza LS, Papafragkakis H, et al. Needle-based confocal endomicroscopy for evaluation of malignant lymph nodes - a feasibility study. Endoscopy 2016;48:923-8. [Crossref] [PubMed]
- Wijmans L, Yared J, de Bruin DM, et al. Needle-based confocal laser endomicroscopy for real-time diagnosing and staging of lung cancer. Eur Respir J 2019;53:1801520. [Crossref] [PubMed]
- Zuo CY, Xue KY, Wu XM, et al. Value of needle confocal laser microendoscopy combined with endobronchial ultrasound bronchoscopy in the diagnosis of hilar and mediastinal lymph node lesions. Kaohsiung J Med Sci 2023;39:936-42. [Crossref] [PubMed]
- Kramer T, Wijsman PC, Kalverda KA, et al. Advances in bronchoscopic optical coherence tomography and confocal laser endomicroscopy in pulmonary diseases. Curr Opin Pulm Med 2023;29:11-20. [Crossref] [PubMed]
- Hariri LP, Mino-Kenudson M, Applegate MB, et al. Toward the guidance of transbronchial biopsy: identifying pulmonary nodules with optical coherence tomography. Chest 2013;144:1261-8. [Crossref] [PubMed]
- Shostak E, Hariri LP, Cheng GZ, et al. Needle-based Optical Coherence Tomography to Guide Transbronchial Lymph Node Biopsy. J Bronchology Interv Pulmonol 2018;25:189-97. [Crossref] [PubMed]
- Ishiwata T, Yasufuku K. Artificial intelligence in interventional pulmonology. Curr Opin Pulm Med 2024;30:92-8. [Crossref] [PubMed]
- Cold KM, Xie S, Nielsen AO, et al. Artificial Intelligence Improves Novices' Bronchoscopy Performance: A Randomized Controlled Trial in a Simulated Setting. Chest 2024;165:405-13. [Crossref] [PubMed]
- Yan S, Li Y, Pan L, et al. The application of artificial intelligence for Rapid On-Site Evaluation during flexible bronchoscopy. Front Oncol 2024;14:1360831. [Crossref] [PubMed]
Cite this article as: Dollin Y, Munoz Pineda JA, Sung L, Hasteh F, Fortich M, Lopez A, Van Nostrand K, Patel NM, Miller R, Cheng G. Diagnostic modalities in the mediastinum and the role of bronchoscopy in mediastinal assessment: a narrative review. Mediastinum 2024;8:51.